Under extreme CO2 radiative forcing (greenhouse effect), built up over millions
of years because CO2 consumption by silicate weathering is slowed by the
cold, while volcanic and metamorphic CO2 emissions continue unabated.
In the 1960's, the climate physicists who discovered the "white
earth" instability knew of no way the climate could recover, short of
waiting for billions of years of Solar evolution. They did not know about
plate tectonics, a concept that revolutionized the earth sciences in the late
1960's. Plate tectonics accounts for the volcanism that delivers CO
2
to the atmosphere and oceans. It also accounts for the flow (subduction) of
CaCO
3 and organic matter into the Earth's mantle, where it is heated
and transformed back into the CO
2 which exits volcanoes. This is known as
the geochemical carbon cycle and a key step in the cycle is the conversion
of CO
2 (as carbonic acid rain) into Ca
2+ and HCO
3- (bicarbonate) ions through
the breakdown ("weathering") of common silicate rocks like basalt.
Silicate rock
weathering consumes CO
2 through chemical reactions that are
temperature and moisture dependent. If global temperatures warm, weathering
rate goes up as does the consumption of CO
2. Conversely, if global climate
gets colder, weathering rate goes down as does the consumption of CO
2. The
temperature dependence acts as a brake, or self-stabilizing mechanism, on
the climate system. But like a truck with bad brakes, it cannot prevent the
Earth crashing into a snowball earth because the braking (silicate weathering
feedback) takes on the order of a million years to take effect. In contrast,
the radiative consequences of ice sheet growth are felt in a matter of months.
Fortunately for us, the slow-acting feedback won out in the end.
In the event of a snowball earth, plate tectonics would continue uninterrupted.
Plate tectonics is driven by the sinking of 100-km-thick slabs of cold rock
under the influence of gravity and does not care if a measely 1.0 km of ice
is floating on the ocean. Consequently volcanism will continue unabated and
more than 100 million years worth of CaCO
3 and organic matter in sea-floor
sediments would be available for CO
2 generation at depth. On the
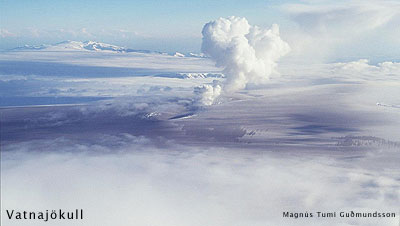
other hand,
if the continents are largely ice-covered and no water left the atmosphere
in the form of rain, silicate weathering would be severely limited to areas
of wet-base ice with neutral
pH. The rate of CO
2 consumption would plummet
while the emissions would remain the same. CO
2 would enter the ocean via sub-sea
volcanoes and vents, and the atmosphere from terrestrial subglacial volcanoes
like those under the present
Vatnajökull in Iceland. On a geological
time scale, CO
2 in the ocean and atmosphere would maintain equilibrium through
air-sea gas exchange in sea-ice cracks and leads (if present). Slowly, the
atmospheric CO
2 must build up due to an imbalance between outgassing and consumption.
The only process that could prevent this is permanent deposition of CO
2 ice
(dry ice) in polar caps. This might limit the attainable CO
2 concentration
below that needed to overcome the surface albedo. The condensation temperature
of dry ice rises with CO
2 concentration (partial air pressure), so there is
a trade-off as CO
2 and consequently temperature rise. Calculations indicate
that dry ice would indeed condense in the polar regions in winter, but that
it would sublimate completely in summer so no lasting "sink" for
CO
2 would exist in polar caps.
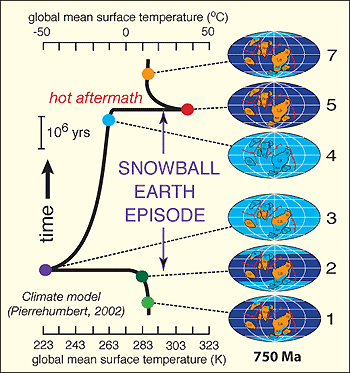
The steady build up of CO
2 will increase the radiative forcing due to the
greenhouse effect, more rapidly at first because of the non-linear dependence
on the CO
2 concentration (i.e., approximately equal response to each CO
2 doubling).
Eventually, the CO
2 radiative forcing begins to rival the radiative loss by
the reflective surface (planetary albedo) and temperatures at the equator
touch the melting point. Surface melt water darkens the ice causing more Solar
radiation to be absorbed and soon areas of open water are exposed, which absorb
radiation with 90% efficiency (versus 60% for bare ice and 10% for fresh snow).
Now the ice-radiative (ice-albedo) feedback works in reverse and combined
with other feedbacks (e.g., ice-elevation feedback: melting lowers the surface
elevation of a grounded ice-sheet, increasing the melting rate due to the
warmer surface air temperature) it drives a cataclysmic meltdown of the snowball
earth. Climate modeling suggests that the meltdown could occur in as little
as 2000 years, which would increase the global runoff by a factor of 10 assuming
2 km average ice cover on the continents and 0.4 km on the ocean. This would
result in a transient stratification of the ocean, in which low-density oxic
meltwater subject to surface warming caps high-density cold brine evolved
for millions of years beneath an ice cover. It would also cause a rapid sea-level
rise and many shelves and platforms that stood in shallow water before the
glaciation would be deeply flooded due to tectonic subsidence during the long
glacial period. Carbonate and silicate rock weathering would accelerate as
the ice retreats, exposing a landscape of rock debris and "flour" from
the grinding action of the ice. Chemical reactions would be hastened by surface
warming, acidic rain and the large surface area of fresh rock available. But
even with accelerated weathering rates it would take tens of thousands of
years to lower the atmospheric CO
2 to a steady state with the now ice-free
surface. Accordingly, the immediate aftermath of a snowball earth is an ultra-greenhouse
transient. In his 1992 paper introducing the snowball earth concept,
Joe Kirschvink
predicted that evidence would be found in the sedimentary record of sudden
switching from glacial to hothouse conditions, and that these changes would
be seen globally. The
1998 paper by the Harvard University group linked this
climate switch to post-glacial "
cap carbonates", observed globally
after the Sturtian and Marinoan glaciations.