No snowball earths have occurred since the first appearance of
bilaterian
animals (above sponge-grade) in the fossil record ~555 Ma in Arctic
Russia. Vascular land plants and terrestrial fauna did not evolve for another
100
plus million years. These species never had to survive a snowball earth.
But a host of microscopic organisms, both
prokaryotes (
archea and
bacteria,
including
prokaryotes (
cyanobacteria)
and
eukaryotes (
algae,
testate amoebae and other protists), and a handfull of cm-scale organisms
(the coiled
Grypania,
1.9 Ga; the necklace-like colonial organism of tissue-grade organization
Horodyskia,
1.5 Ga; the worm-like Parmia, 1.0 Ga), evolved before the
Sturtian
and
Marinoan snowball
earths and survived. Evolutionary rates appear to have been incredibly slow,
however, compared with post-snowball times, and
this
has
discouraged
intensive investigation of the fossil record. Palynofloras were impoverished
during the
Cryogenian
Period (encompassing the Sturtian and Marinoan snowball
earths), relative to earlier and later periods, but the characteristic assemblage
of simple thin-walled microspheres (
leiospheres)
passed through the Marinoan snowball earth without change according to
Kath
Grey, the leading specialist
in the field, at
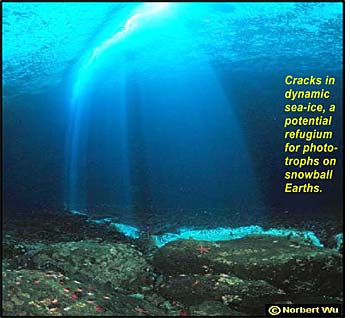
the Geological Survey of Western Australia in Perth. Similar
palynofloras were earlier reported from Sturtian glacial deposits in East
Greenland and Utah (USA). Several important
eukaryotic lineages
from more ancient times, including red, brown and
chromophyte algae,
are still extant and must have survived. This implies that sunlight and
liquid water coexisted
somewhere on the snowball earths. The liquid water need not have been extensive
or permanent, snow algae need only a surface film of water, and a dash of
dust, when the snow touches the melting point in summer. But Kath Grey's
data from Western Australia suggest the need for a marine refugium in the
snowball earth. This is supported by organic molecular fossils (biomarkers)
diagnostic of
phototrophic bacteria
and eukaryotes recently described by a
group led
by
Alison
Olcott of the University of Southern California (USA)
from within glacial marine sediments in Brazil. A necessary qualifier is
that most glacial sediments that survive were deposited during the glacial
retreat, when open water was present irrespective of the earlier ice extent.
The survival of
photoautotrophy has inspired investigation of sea-ice dynamics
in a snowball earth utilizing computational climate models. With mean surface
temperatures of -50°C (-74°F), floating ice thickens rapidly to ~1.0 km and flows
under its own weight towards the equator, where it is thinnest and where ice
inflow is balanced by melting and
sublimation. Flowage of the floating "sea
glaciers" causes cracking along ice-grounding lines (separating free-floating
ice and grounded ice). The cracks are held open by the force of the water, allowing
them to be healed by new sea ice, which is full of brine channels which drain
away in a few years.
Brine channels in present-day
sea ice host a
surprisingly
rich biota including algae (mostly diatoms), protozoa and foraminifera. The biota
must be tolerant of hyperoxia because photosynthetic O
2 cannot escape the brine
channels. This might be an interesting pre-adaptation if atmospheric O
2 levels
rose after the Marinoan snowball earth, which was a necessary prerequisite for
the rise of large active animals.
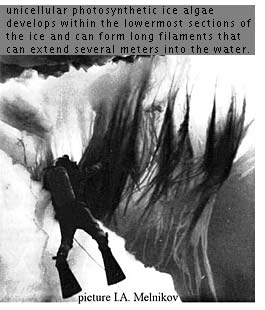
Early snowball models with sea-ice dynamics yielded tropical ice far too thick
(>100 m) for photosynthesis. However a recent
model result by Dave Pollard
and Jim Kasting at the Pennsylvania State University (USA) yields a 2000-km-wide
equatorial zone where the ice is less than 2 m thick, alongside km-thick ice
poleward of ~13° degrees latitude north and south. The steep transition zone
closely follows the snow line on the moving ice. The result is stable only if
the equatorial is clear (old) sea ice, which absorbs Solar radiation better than
bubbly ice formed from compacted snow. A healthy rate of photosynthesis is possible
under 2 m of clear ice (the shut-off is ~20 m). In addition, 2-m-thick ice (similar
to present annual sea ice) would have abundant cracks and leads, where marine
photosynthesis would flourish given dust (nutrient iron, phosphorus and trace
elements) and fixed nitrogen. Snowball climate models indicate that the subtropics
(below the descending arms of the atmospheric
Hadley cells) would experience
net
sublimation, meaning that subtropical land areas remote from mountains would
remain ice free and generate dust. Volcanic ash and extraterrestrial material
would contribute regionally and globally, respectively, to the dust load.
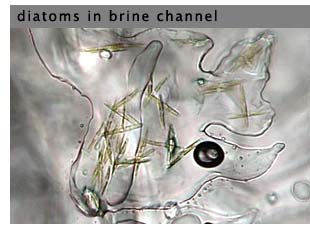
Climate model results have been reported by
Dick
Peltier at the University of
Toronto and associates in which a megacontinent is glaciated from pole to equator
while large areas of the ocean remain as ice-free "oases". This model
is minimally challenging to life but less satisfactory in accounting for the
geochemical anomalies associated with the glacial deposits, or with the apparent
duration of the glacial episodes (read:
What is the evidence for the snowball
earths?). The paleogeography used, dominated by a polar supercontinent, is completely
different from that implied by paleomagnetic data (read:
What
caused the snowball
earths?), making it difficult to evaluate the model results. Both the oasis
and
thin-ice ice soloutions lapse to thick tropical ice (aka "hard" snowball
earth) with modest lowering of radiative forcing. It is disquieting to believe
that the survival our eukaryotic ancestors depended on the narrow-escapes offered
by these metastable states.