Possibly by a lowering of atmospheric greenhouse gases to near-present levels
through tectonically-mediated rock weathering, when the Sun was considerably
dimmer than present.
Evidence points to a reduction in so-called "greenhouse" gases in
the atmosphere, principally CO
2 (carbon dioxide) and CH
4 (methane).
This would have made the global climate colder, creating larger areas of ice
and snow.
Ice and snow reflect more Solar radiation than does bare ground or liquid water,
which creates a "positive feedback". If the earth ever became approximately
half-covered by ice or snow, the feedback would become self-sustaining and glacial
ice would rapidly spread to the equator.
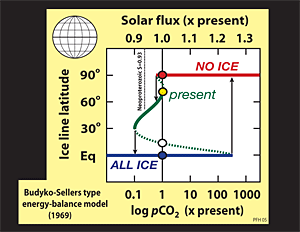
Climate physicists (notably
Mikhail
Budyko in Leningrad, USSR and William Sellers in Tucson, Arizona, USA) accidentally
discovered the runaway ice-albedo feedback in the 1960's as a result of
calculations (using simple radiative energy-balance models) concerning the stability
of Arctic sea-ice. The physicists did not believe a runaway feedback had ever
actually occurred. They were unaware that a geologist,
Brian
Harland at Cambridge
University in the UK, was marshalling geological evidence for global glaciation.
(Harland was equally unaware that physicists had an explanation for his observations.)
The physicists assumed that life could not have survived a snowball earth and
they knew of no way the planet could have escaped from the icy grip. The subsequent
discoveries of deep-sea and
hydrothermal-vent biotas
and Antarctic
psychrophiles
(cold-loving organisms) removed the first objection, and in 1992
Joe Kirschvink
(read:
How did the snowball earths terminate?) postulated a natural escape mechanism
involving CO
2.
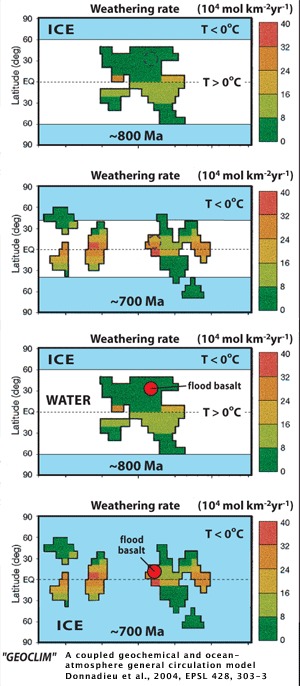
What caused the lowering of greenhouse gas concentration in the first place?
The scenarios for CO
2 and CH
4 are quite different, and each has been advocated
as an agent of snowball earth. First CO
2. On geological time scales, the ocean
and atmosphere are in equilibrium with respect to CO
2 and can be treated as
a single reservoir. CO
2 is supplied to this reservoir by volcanic and metamorphic
emanations, and is removed as sediment in the form of CaCO
3 (limestone) and
organic matter (roughly CH
2O). The atmospheric CO
2 forms carbonic acid rain,
which is neutralized (protons are consumed) by silicate rock "
weathering" (conversion
to soil). The resulting solutes include Ca
2+ and HCO
3- (bicarbonate) ions that
rivers carry to the ocean, where CaCO
3 is precipitated by calcifying organisms
and organic matter by primary producers like
cyanobacteria and
algae. The entire
process is often simply referred to as "silicate weathering", because
that is the rate-limiting step. Silicate weathering rate is sensitive to climate,
faster where hot and wet, slower where cold and dry.
During the
Cryogenian Period, encompassing the Sturtian and Marinoan snowball
earths, there was a rare preponderance of continents in the tropics, where it
is hot and wet. Therefore, the global rate of silicate weathering was high.
As a result, CO
2 concentrations fell and the global climate cooled
because there was less "greenhouse" warming. Global cooling lowered
the silicate weathering rate, ultimately stabilizing the climate system at a
new colder state.
Two additional phenomena are known to have occurred at the same time which further
contributed to high silicate weathering rates and therefore a cold climate.
The first was the breakup of a pre-Pangean supercontinent named Rodinia, which
began ~830 Ma and continued for nearly 200 million years. A supercontinent is
the assembly of almost all continents into a single mass. Silicate weathering
rates are low when a supercontinent exists, because most land area is far from
the ocean and therefore very dry. When a supercontinent breaks up into small
fragments, formerly arid regions become wetter and weathering rates increase
accordingly. The second phenomenon was the massive eruption of basalt lava ("flood" basalt)
at 723 Ma in Arctic Canada, which was then very close to the equator. Basalt
lava weathers rapidly and is a rich source of Ca
2+ ions. The combined effects
of tropical continents, supercontinent breakup, and equatorial flood basalt
emplacement are sufficient to cause a snowball earth in model simulations published
in 2004 by Yannick Donnadieu, Yves Goddéris and coworkers.
Scenarios involving CH
4 are simpler but more ad hoc. CH
4 is
supplied to the atmosphere by microbes (
methanogens)
that live in poorly-drained soils (e.g., tropical wetlands) and in organic-rich
sediments below the sea-floor. It is
removed by oxidation (combination with O
2 or its derivitives). Molecule
for molecule, CH
4 is ~30 times more powerful as a greenhouse gas
than CO
2, but it
is very unstable in our present O
2-rich atmosphere where its "residence" time
is ~20,000 times less than CO
2. O
2 levels in the early
atmosphere were extremely low (less than 1% PAL, present atmospheric level)
and CH
4 levels were presumably
far higher than present, creating a strong greenhouse effect. The early CH
4
greenhouse helped offset the lower Solar luminosity, which has increased by
an average of ~6% per billion years since the origin of the Solar System. When
O
2 levels rose, CH
4 levels fell accordingly, causing a
loss of greenhouse warming. If the loss was rapid (less than 1 million years),
the cooling could not be
stabilized by silicate weathering feedback, which would cause a slow compensatory
rise in CO
2. If the previous CH
4 greenhouse forcing was
large, a snowball earth would result from its rapid destruction.
The most likely cause of a sudden rise in O
2 is the evolution of
oxygenic photosynthesis (a biological revolution), but preliminary evidence
form fossil organic molecules
suggests that oxygenic photosynthesis was in existence half a billion years
before the Makganyene snowball earth. Different geochemical data-sets suggest
that the rise of O
2 above 1% PAL occurred sometime between ~2.4 and
2.2 Ga (billion years ago). Resolving the detailed trajectory of O
2 rise
and its relation to the Makganyene snowball earth is an active area of current
research.
Various astronomical theories for triggering snowball earths have been proposed.
In 2005, Alex Pavlov and associates
suggested that snowball earths occurred
when the Solar System encountered giant molecular clouds in the spiral arms
of our galaxy. An isotopic test of their hypothesis has yet to bear fruit.