Kirschvink responded to the positive Elatina fold test with a theory he
first aired in 1989 (Maugh, 1989). It eventually appeared as an article,
seven paragraphs long, embedded in a 1348-page book (Kirschvink, 1992).
It postulated that conditions amenable to global glaciation were set up
by an unusual preponderance of land masses within middle to low latitudes,
a situation that has not been encountered at any subsequent time in Earth
history. One effect of such a paleogeography would be a substantially higher
albedo in the sub-tropics, where clouds are least important (Kirschvink,
1992). Any resultant glaciation would further increase the Earth's
albedo by lowering sea level, exposing continental shelves and inland seas
(Kirschvink, 1992). Placing more continents in the tropics would also increase
the silicate weathering rate (provided tectonic uplift was not mysteriously
absent there), leading to a colder planet with lower atmospheric pCO
2 (Marshall
et al., 1988; Worsley and Kidder, 1991). In addition, the meridional heat
transport by the Hadley cells would be weakened because tropical air masses
would be drier on average with greater continentality. Oceanic heat transport
might also be lessened, but this is less certain. These combined effects
might lead to the growth of large ice caps, nucleated on islands or continents
bordering the polar seas.
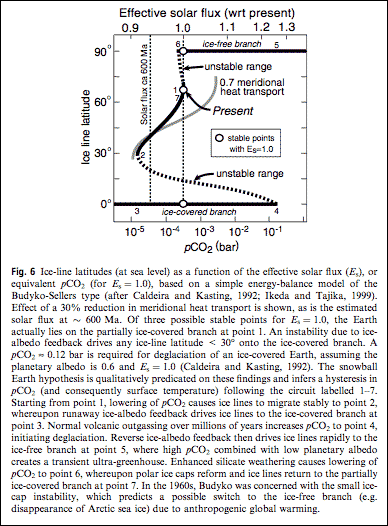
Ice caps create a positive feedback on climate change through ice-albedo
feedback (Croll, 1867; Budyko, 1966; Manabe and Broccoli, 1985). Early
energy-balance climate models revealed a fundamental instability in the
climate system caused by this positive feedback (Eriksson, 1968; Budyko,
1969; Sellers, 1969; North et al., 1981), an effect reproduced in a number
of GCMs (Wetherald and Manabe, 1975; Jenkins and Smith, 1999; Hyde et al.,
2000; Pollard and Kasting, 2001). If more than about half the Earth's
surface area were to become ice covered, the albedo feedback would be unstoppable
(Fig. 6). Surface temperatures would plummet (Fig. 7), and pack ice would
quickly envelope the tropical oceans (Hyde et al., 2000; Baum and Crowley,
2001; Warren et al., 2002). With reduced Neoproterozoic solar forcing,
the ice-albedo instability occurs in the GENESIS v2 GCM between 1.0 and
2.5 times modern pCO
2 with different paleogeographies (Baum and Crowley,
2001; Pollard and Kasting, 2001). The time scale of the sea-ice advance
is uncertain—the ocean's thermal inertia resists the ice advance
initially (Poulsen et al., 2001), but the ocean is a finite heat reservoir
and cooling over thousands of years would leave it powerless to resist
the ice encroachment. It is also uncertain if the tropical ocean would
ever become entirely ice covered (Hyde et al., 2000; Baum and Crowley,
2001)—Kirschvink (1992) speculated that areas of open water (polynyas)
would remain, tracking the zone of highest solar incidence back and forth
across the equator and imparting a strongly seasonal climate even at low
latitude, consistent with geological observations (Williams and Tonkin,
1985). This is distinct from the tropical "loophole" model
(Hyde et al., 2000; Baum and Crowley, 2001; Crowley et al., 2001), in which
the ice fronts miraculously approach but never cross the ice-albedo instability
threshold [but the continents are glaciated because they are mostly placed
in middle and high latitudes, contrary to paleomagnetic evidence (Evans,
2000)].
Assuming an albedo runaway did occur, the climate would be dominated by
the dry atmosphere and the low heat capacity of the solid surface (Walker,
2001). It would be more like Mars (Leovy, 2001) than Earth as we know it,
except that the greater atmospheric pressure would allow surface meltwater
to exist. Diurnal and seasonal temperature oscillations would be strongly
amplified at all latitudes because of weak lateral heat transfer and extreme "continentality" (Walker,
2001). Despite mean annual temperatures well below freezing everywhere,
afternoon temperatures in the summer hemisphere would reach the melting
point (Walker, 2001). Evaporation of transient melt water would contribute,
along with sublimation, to maintain low levels of atmospheric water vapor,
and glaciers would feed on daily updrafts of this moisture (Walker, 2001).
The global mean thickness of sea ice depends strongly on sea-ice albedo
(~1.4 km for albedo 0.6) and meridional variability is a complex function
of solar incidence, greenhouse forcing (see below), zonal albedo, ablation
or precipitation, and equatorward flowage of warm basal ice (Warren et
al., 2002).
Climate physicists originally assumed that no ice-albedo catastrophe ever
actually occurred because it would be permanent: the high planetary albedo
would be irreversible. A saviour exists, however, and Kirschvink (1992)
identified it as the buildup of an intense atmospheric CO
2 greenhouse through
the action of plate tectonics in driving the long-term carbon cycle (Walker
et al., 1981; Kirschvink, 1992; Caldeira and Kasting, 1992). On a snowball
Earth, volcanoes would continue to pump CO
2 into the atmosphere (and ocean),
but the sinks for CO
2—silicate weathering and photosynthesis—would
be largely eliminated (Kirschvink, 1992). Even if dry ice condensed at
the poles in winter, it would likely sublimate away again in summer (Walker,
2001). CO
2 levels would inexorably rise and surface temperatures would
follow, most rapidly at first and more slowly later on (Fig. 7) due to
the non-linear relation between CO
2 concentration and the resultant greenhouse
forcing (Caldeira and Kasting, 1992). With rising surface temperatures,
sea ice thins but ground ice sheets expand in some areas due to a stronger
hydrologic cycle. If CO
2 outgassing rates were broadly similar to today
(a reasonable assumption for 600-700 Ma), then the time needed to build
up the estimated 0.12 bar CO
2 required to begin permanent melting at the
equator, assuming a planetary albedo of 0.6, would be a few million years
(Caldeira and Kasting, 1992; Crowley et al., 2001). This estimate (Fig.
7), while subject to large uncertainties, is of the same order as the estimated
time-scale of LNGD from paleomagnetic (Sohl et al., 1999) and stratigraphic
(Hoffman et al., 1998a) evidence. Once the tropical ocean begins to open
up perennially, deglaciation proceeds rapidly due to reverse ice-albedo
feedback (Caldeira and Kasting, 1992; Crowley et al., 2001). The fall in
planetary albedo occurs far faster than the excess atmospheric CO
2 can
be consumed by silicate weathering, with the result that a transient heat
wave must follow the ice retreat (Kirschvink, 1992; Caldeira and Kasting,
1992). Kirschvink (1992) concluded that a cold planet with many tropical
continents and large polar sea-ice caps "would be a rather unstable
situation, with the potential for fluctuating rapidly between the 'ice
house' and 'greenhouse' states".